Baker’s yeast teaches us how to budget during a crisis
Article and artwork by Preethi Ravi
Image: Control of metabolic specialization by Aspartate
They brew your beer and bloat your bread. They inhabit the skin of fruits and colonize some of the deepest parts of the human body.
Say hello to the whiz kid of the fungal kingdom, Saccharomyces cerevisiae, commonly referred to as baker’s yeast.
Highly versatile and quick to replicate, colonies of yeast are microscopic factories that primarily run on glucose. However, glucose in the natural environment is often a premium, but meager resource. How then do these cells flourish and pull off such massive projects? It seems that yeast do this by forming a community of millions of cells that share resources and earmark populations for distinct tasks. Now, who dictates the roles and responsibilities in such densely populated communities? How do cells develop a ‘chemistry’ amongst themselves?
A team of researchers from inStem unbox the answers to these questions. In the recent paper published in eLife, Sunil Laxman and his group identify that yeast cells, when starved of glucose, self-organize into distinct populations by making use of a bountiful amino acid resource called aspartate. The populations that emerge are genetically identical, but have contrasting metabolic signatures. This is because aspartate, being a malleable biomolecule, lends itself as a carbon source to one population and nitrogen source to the other. Such an efficient budgeting of aspartate demarcates the biological duties of the two clusters, which then join forces to form a well-structured community. In fact, the authors believe that these findings made in yeast could have implications in how we look at multicellularity in stem cells, cancer cells and microbial colonies.
“This is one of the first studies to demonstrate this idea of varying 'demand and supply' in different cells, even though they are genetically identical and in the same environment,” says Laxman.
Yeast cells, when sapped of glucose, turn on a metabolic reaction called gluconeogenesis where the end product is trehalose (made of two glucose molecules). When trehalose levels hit a tipping point, it coerces the clonal yeast cells to diverge into two distinct metabolic populations. This was discovered in an earlier study by the same group wherein they found that these two populations formed a spatially organized ‘floret’ structure; with the inner region of dark cells carrying out gluconeogenesis, and the outer petals made of light cells carrying out glycolysis by consuming trehalose.
“Taking off from the previous work, I wanted to figure out how these cells were making a limiting resource such as trehalose under low glucose conditions. Who is providing them the carbon backbone if glucose is scant?” asked Sriram Varahan, lead author of both the studies.
The search for these answers was spurred by the fact that low glucose conditions are often blessed with copious amounts of amino acids. Hence Sriram suspected that amino acids could be fuelling gluconeogenesis in situations where glucose levels were exiguous. So, by meticulously tracking and quantifying the flow of carbon through the gluconeogenic cycle, he found that trehalose indeed derived its carbon backbone from amino acids and exclusively from aspartate.
“Aspartate is a nitrogen source that is important for nucleotide synthesis. This finding brings into light an underappreciated role of aspartate as a carbon source that also drives gluconeogenesis,” explains Sriram.
Although there is a generous supply of aspartate, not all cells in the colony flush this amino acid into gluconeogenesis. Only some cells steer the flux of aspartate into trehalose, thereby spawning metabolic heterogeneity in the population. How does aspartate get disbursed among the cells?
A rewarding collaboration with Sandeep Krishna’s lab seeded some ideas. Through this partnership, they modelled this division of labor, where dark cells predominantly used aspartate as a carbon source to make trehalose. On the other hand, light cells used it as a source of nitrogen to whip up nucleotides. Thus the ‘plasticity’ offered by aspartate created a nifty carbon-nitrogen budgeting plan for the two populations.
Curiosity led them to find out how this benefitted the community during a crisis. It turns out that the dark gluconeogenic cells steel the colonies from stressors such as desiccation and freezing; while the light cells, promote the expansion of the colony in search of nutrients.
When discussing the possible implications of these findings, Sriram feels that the experimental conditions used in this study are quite similar to a number of scenarios where glucose is limited but amino acids are a plenty. “Many parallels can be drawn between these colonies and say for example, tumors. Although genetically identical, cancerous cells at the core of a tumor have different metabolic characteristics, compared to cells at the periphery. Identifying these cell states could help understand tumor progression,” he elaborates.
Yeast cells are indeed great teachers, if one asks the right questions. This group most certainly did.
About the Researchers:
Sunil Laxman is a faculty at Regulation of Cell Fate (RCF) theme at inStem and an Intermediate Fellow of Wellcome Trust/DBT India Alliance. Sriram Varahan is a Wellcome Trust/DBT India Alliance Early Career Fellow at Dr. Laxman’s Lab. Adhish Walvekar is post-doctoral fellow at the University of Luxembourg, who was formerly a graduate student at Sunil Laxman's lab. Sandeep Krishna is a faculty at the Simons Centre for the Study of Living Machines at NCBS Bangalore, and an adjunct faculty at Niels Bohr Institute and ICTS Bangalore. Vaibhhav Sinha is a grduate student at Sandeep Lrishna's group.
Reference:
Resource plasticity-driven carbon-nitrogen budgeting enables specialization and division of labor in a clonal community, Sriram Varahan, Vaibhhav Sinha, Adhish Walvekar, Sandeep Krishna and Sunil Laxman. eLife 2020;9:e57609, Pub: 2nd Sep, 2020
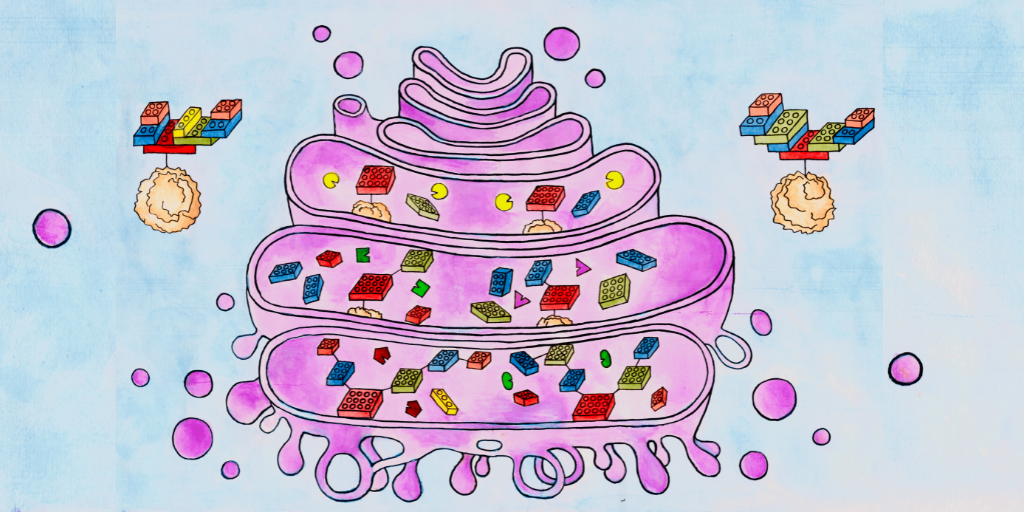
Building Biomolecules, One Step At A Time
- 0comment(s)

Interested in working with us?
- 0comment(s)